Is Low-Level Radiation Good For Us? Interview with Dr. Chris Thome
Dr. Thome's research at the world's deepest clean lab explores how radiation may be critical for normal cell function and genomic stability
Dr. Chris Thome is a radiation biologist and assistant professor at NOSM University. He also works on the REPAIR project I mentioned in my primer on ionizing radiation. I interviewed Dr. Thome on January 5, 2023 to ask him about his research at SNOLAB and the implications it may have on how we understand low dose ionizing radiation.
Madi Hilly: Can you start by describing SNOLAB and the research project you're working on there?
Chris Thome: Sure. Our project is called the REPAIR project. It stands for “researching the effects of the presence and absence of ionizing radiation.” That's essentially what we're doing; we're looking at the effects of the absence of natural background ionizing radiation and how that impacts normal cellular and molecular function within different biological systems.
We're exposed to natural background ionizing radiation all the time. And it comes from a combination of terrestrial and cosmic sources. Pretty much all low-dose radiobiology research is always done in the presence of this very low dose rate from natural sources. What we're interested in looking at is what happens if you actually remove that natural background radiation. It's something that all biological systems have evolved and adapted in the presence of. Our hypothesis is that if you remove natural background radiation, you can actually disrupt some of these normal cellular processes, and it could actually be a detrimental thing to living systems.
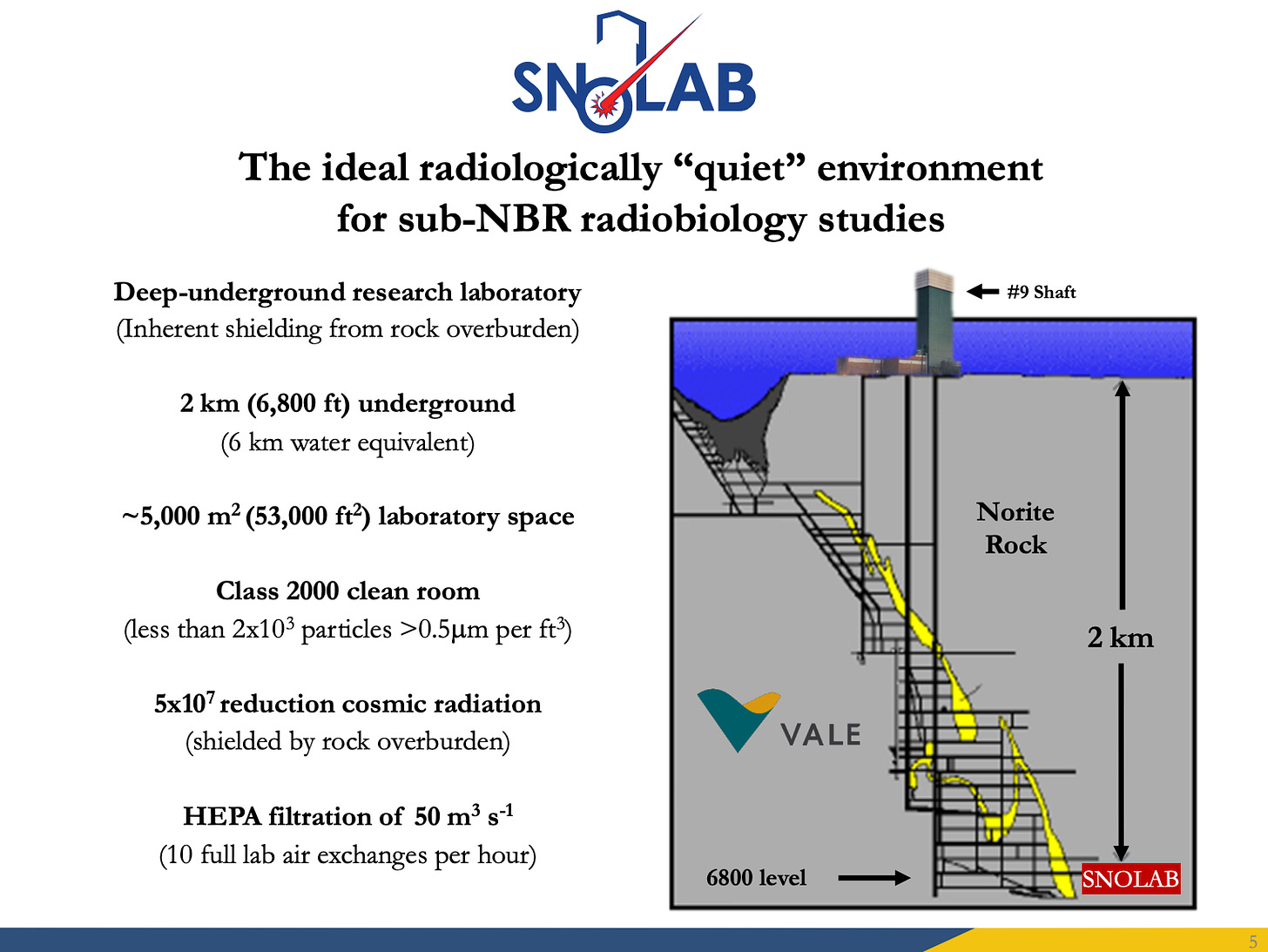
The reason we're doing it in SNOLAB is that some of the background radiation, particularly cosmic rays, are really high energy. It's not practical to design shielding in a normal lab on the surface and be able to get a significant reduction in those cosmic sources. So by going down underground into SNOLAB, we have that two kilometers of overhead rock which basically eliminates all cosmic radiation. That's why that lab was built. It's one of a few labs around the world that are built underground. Most of what they do is astroparticle physics research, looking at things like dark matter and neutrinos. But we're taking advantage of that infrastructure and that overhead shielding to be able to grow biological systems in the almost complete absence of natural background radiation.
MH: When you say almost complete absence, are you still having to do some sort of shielding or filtering for the terrestrial radiation?
CT: Exactly. By going underground, we pretty much get rid of all of the cosmic radiation. The main source of cosmic radiation that we are exposed to on the surface is from particles called muons. And there’s about 50 million times lower muon flux underground compared to the surface. So for all intents and purposes, we're eliminating all of the cosmic sources.
But then we still have the terrestrial sources because the lab is surrounded by rock. We have different isotopes in the rock like uranium, thorium, potassium-40. And so we have some radiation emanating from the rock. One of the main ones we have is radon gas, which is found in basements and in mines. So we have to deal with that.
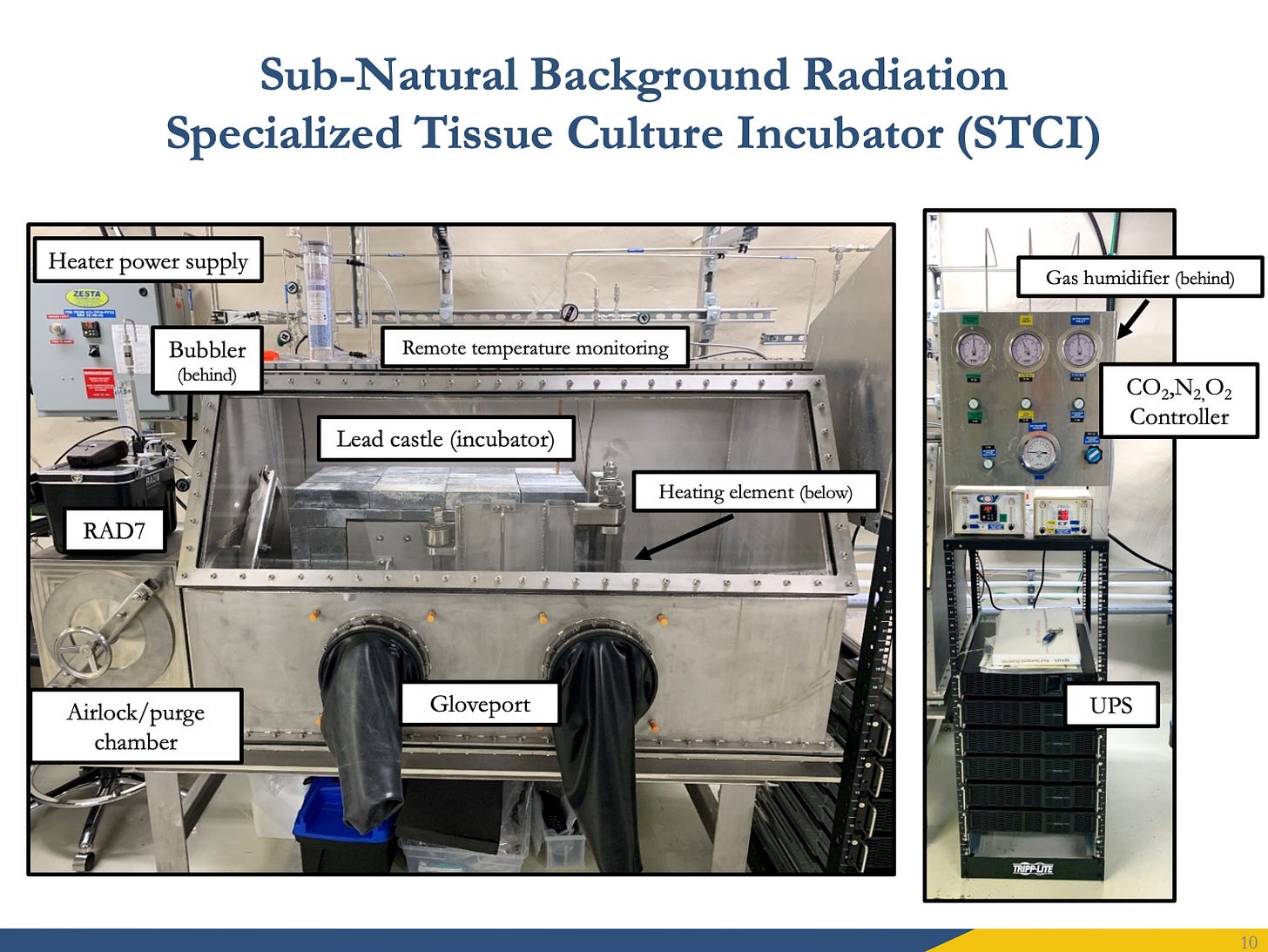
The other thing we have to deal with is that any of these biological systems that we culture have internal radiation within them. They have carbon and potassium in them. Part of that potassium is radioactive potassium-40, part of the carbon is radioactive carbon-14. We're pretty good now at getting rid of almost all of the external sources by going down to SNOLAB and then adding some shielding. But we're still having to deal with some of those internal sources because we can't just remove potassium completely from living systems, and we can't remove carbon from living systems. We're working around that and trying to come up with ways that we can get rid of some of that internal radiation as well.
MH: Wow, that's really interesting. Is that what you're working on right now? How to deal with that internal radiation?
Yes. So going back to the external terrestrial radiation - What we've done is we've designed a special incubator down in SNOLAB, mainly for the radon gas. The nice thing about radon is that it does have a short half life of 3.8 days. What we've done is create an incubator that's a completely enclosed glove box that we feed with air from compressed gas cylinders. And if we fill them with air from the surface and let them age for a month or two, the radon gas has pretty much decayed away to almost nothing. So we can feed air into this incubator that is almost free from radon gas. That allows us to control for the radon. We've also designed some lead shielding which helps us control from some of the external gamma radiation coming off the rock. Now we're not 100% perfect in that, but we are able to get those terrestrial components – the radon and the gamma radiation – down pretty low.
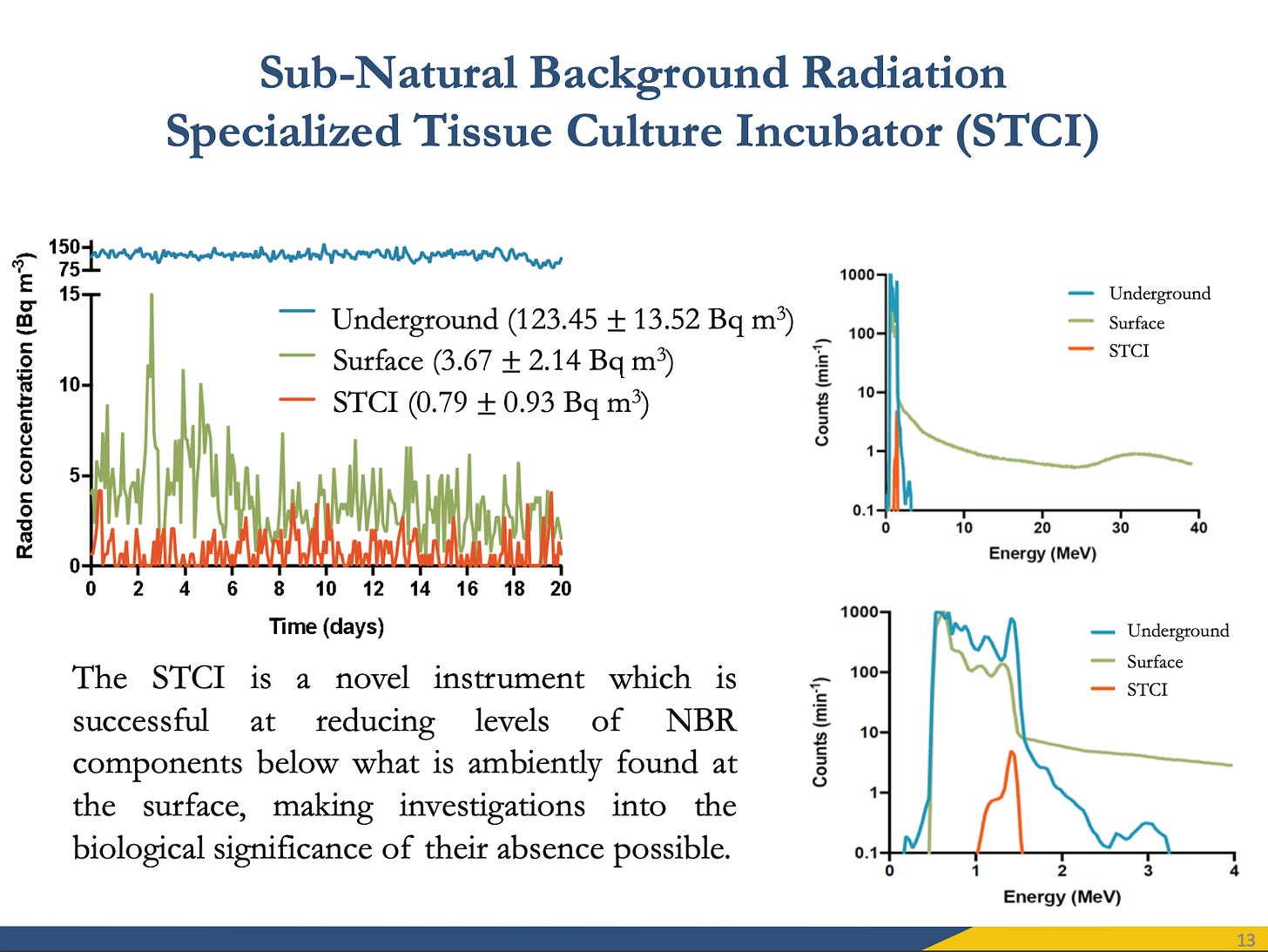
We've done some detailed dosimetry on all of our systems. The carbon-14 is actually not too much of a problem. In terms of the overall background dose rate, it's actually pretty low. But the biggest one for us is the potassium-40. Currently, if we bring down human cells and we grow them in our low-background, glovebox-type-incubator, about 97% of our dose rate right now is coming from potassium-40 within the cells themselves.
What we've done is we've actually sourced out a company that can provide us with a potassium salt that has been enriched in K39, the non-radioactive component. So it has a lower level of potassium-40 compared to what you normally get. Normal potassium is actually very low in potassium-40. About 0.01% of all potassium is potassium-40. But that's still enough to give us a pretty significant dose rate. Now we've sourced out this potassium salt that is enriched in potassium-39 and has lower levels of potassium-40. We can culture our biological systems in that enriched potassium and, over time, they will exchange out a lot of potassium-40 within the cells and take up more of the enriched potassium. This is something we've only just started in the last month or so.
MH: So you're trying to scrub out all external radiation and as much internal radiation as possible. Zooming back out, why? What question are you trying to answer?
CT: This is a question we get a lot. “Why do we care about this? Because humans live on the surface and are exposed to natural background radiation – not underground in caves that are completely shielded from radiation.” So, why is this important?
What we're really trying to do here is just answer the question of “what is the biological effect of low dose radiation in general?” Earlier you mentioned LNT. We’re trying to challenge that linear no-threshold hypothesis down in the low dose region.
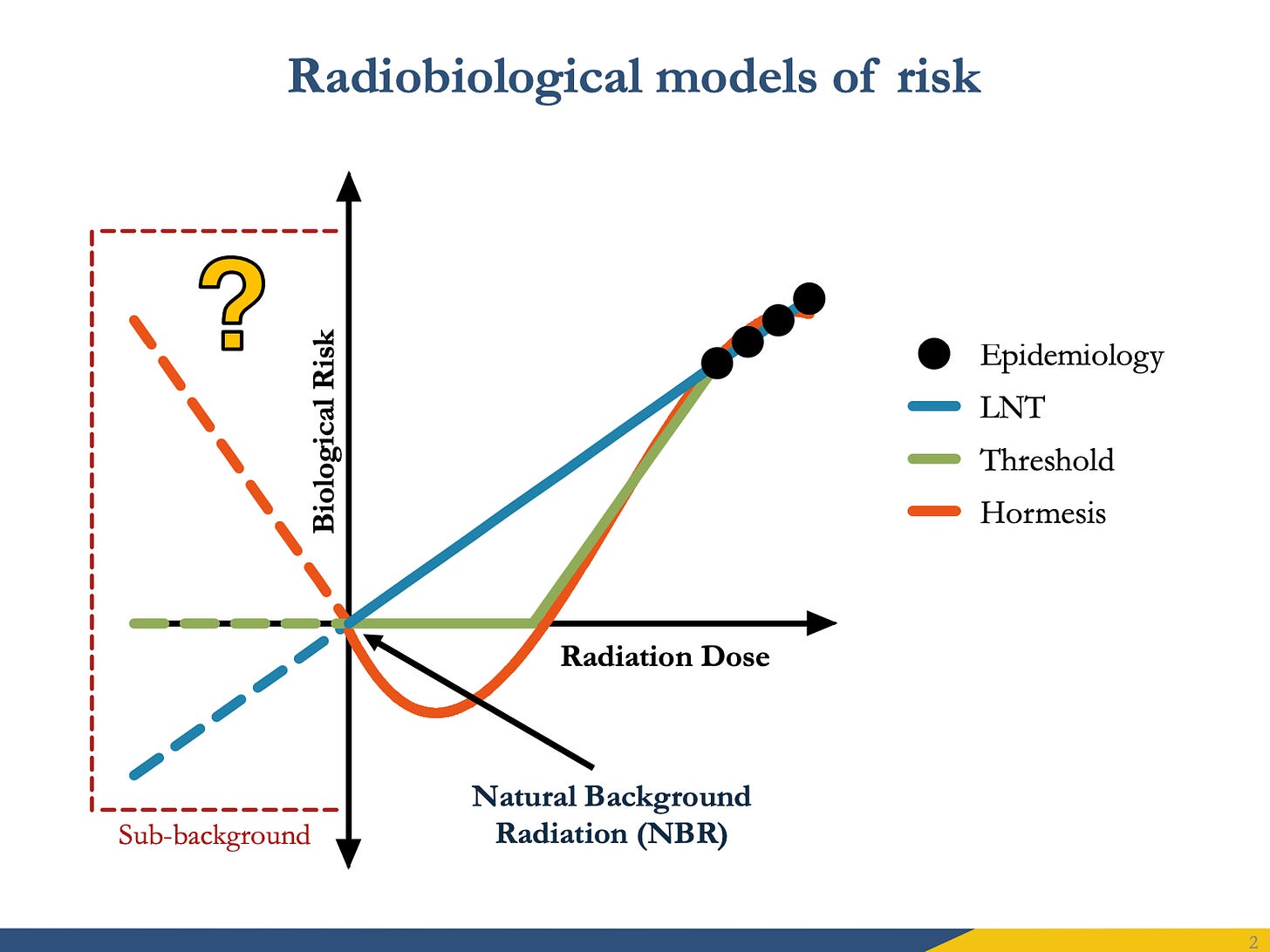
A common approach in biological research when we’re trying to figure out the effect of something is to remove it and we see what the consequences are. For example, we know of many different genes that are involved in cancer. Partly how we know that is by removing those genes from biological systems. If we see increases in cancer rates we know that that gene is important in preventing cancer.
What we're doing here is kind of the same thing. We're trying to understand what role natural background radiation plays in normal cellular function. The way that we're doing that is by removing it. And if we remove it, and we see detrimental effects in our different systems, then we can conclude that natural background radiation is something that's essential for normal cellular function and normal homeostasis in biological systems.
MH: The idea would be that organisms, including humans, are constantly awash in natural background radiation and have evolved in low levels of ionizing radiation. Therefore, it may actually be imperative for life – and the absence of that radiation may be detrimental to health.
CT: Exactly. Getting a little bit more into into why we hypothesized that, we're thinking that the little bit of damage you get from natural background radiation – it's small in comparison to a lot of other types of stressors that we're exposed to – but that little bit of damage keeps things functioning properly. It keeps your things like DNA repair and antioxidant processes active. If you remove it, you might now start to shut down some of these natural processes. And in doing so, now you’re making yourself more susceptible to damage.Basically, by removing that background radiation, we might actually be suppressing some of these beneficial natural processes.
MH: I’ve compared radiation hormesis to weightlifting. Lifting way too much can kill you. Lifting a little less but still too much can injure you. Lifting the right amount leads to maximum gains and good muscle strength. And no resistance exercising or just sitting on the couch all day leads to really weakened muscles and worse health outcomes. Is that sort of the idea? A little bit of damage or stress keeps the repair mechanisms functioning?
CT: That’s pretty much it. You know there’s the saying that a little bit of everything in moderation is good for you. The same could be true with radiation.
We talk a lot about DNA repair and antioxidant processes, but radiation has effects on the immune system as well. So it could be all these things combined. A little bit of radiation could actually help to keep these processes functioning.
MH: If radiation hormesis is proven – if a little bit is in fact good for you – what would the real world implications be? Is it that we should all be more chill about radiation, or does this unlock how we tackle diseases?
CT: Yes, I think both of those. Long-term, we could actually use this in treatment of disease. And it is something that is being done currently, not so much in North America but particularly in Europe. In Germany for example, they use low-dose radiation and treatment for some benign medical conditions – things like arthritis, autoimmune diseases, and inflammatory diseases. We've actually just completed a clinical trial looking at using low-dose radiation as a way to treat recurrent prostate cancer. And we did see some success in that.
I mentioned the effects of radiation on the immune system. There is some evidence out there that low dose exposures could actually stimulate immune function. We could use this as a kind of immunotherapy to treat cancer and other diseases.
This research was done a lot back in the ‘60s and ‘70s. When fear of radiation started to increase, it kind of fell out of practice. But we've started looking back into that a little bit. And there's still a lot we need to know in terms of optimal doses, dose rates, timing of delivery, the mechanism of what specific immune cell types are being impacted. So this is more long-term, but it could actually be something that we use therapeutically to treat disease.
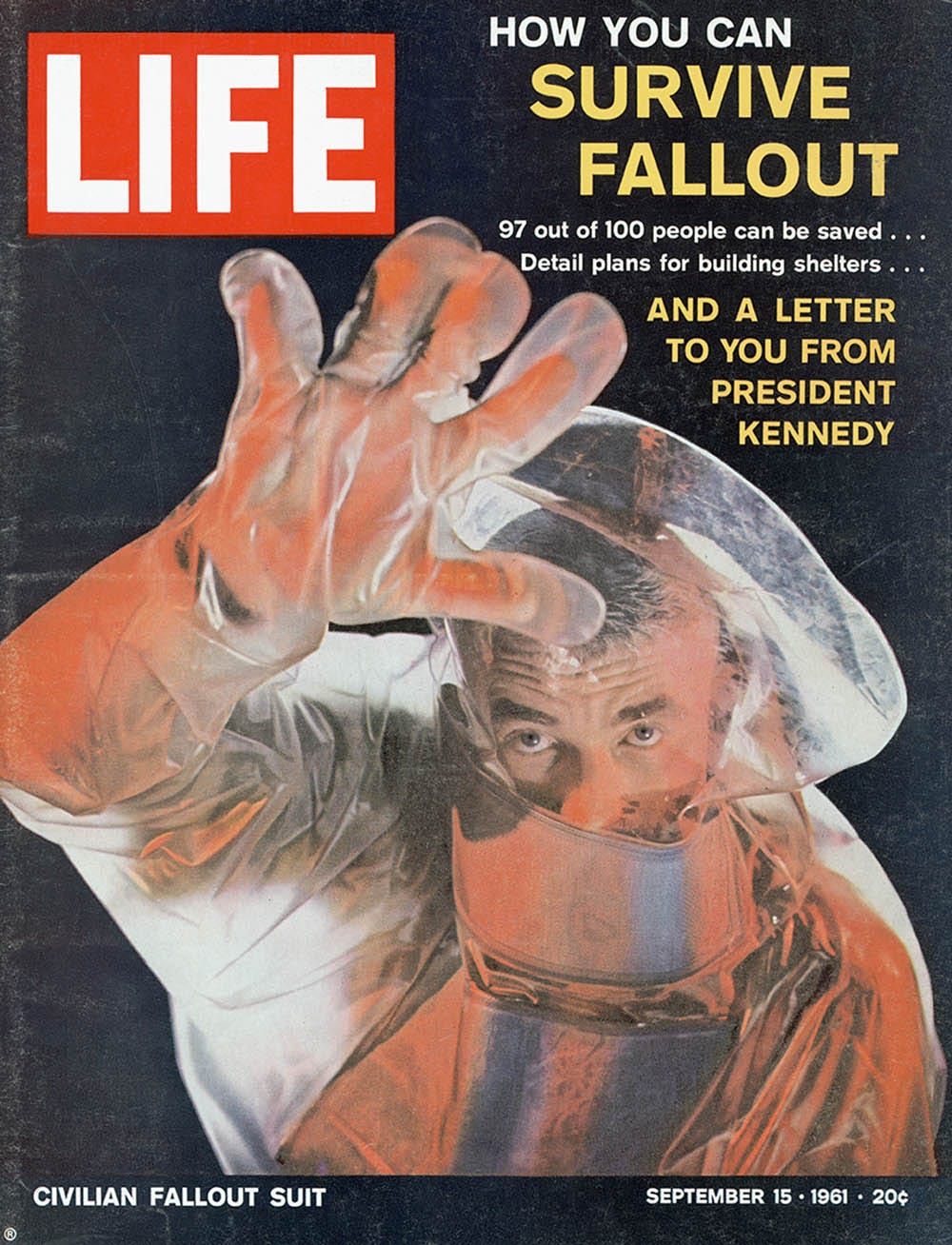
But I think more importantly, the short-term implications of it like you said would be to reduce people's fears around radiation exposure. It’s important for members of the general public, but also for industry. The nuclear industry is one of the most highly-regulated industries in the world. Think about the amount of money they spend on radiation protection based on LNT. If some of the data does not support that model of radiation risks, that could have impacts on radiation protection standards.
The same could apply to medical practice as well. We see that some physicians are hesitant to prescribe procedures like CT scans because of the radiation exposure. So it could have impacts on overall perception of radiation exposure from these low-dose sources, medical or occupational.
MH: You've mentioned a few of the areas of research REPAIR has looked into. What in your opinion has been the most important finding to date? What about your most surprising finding?
CT: We're in the midst of a lot of different experiments. We're experimenting on different human cell lines, where we can culture them in petri dishes and flasks.
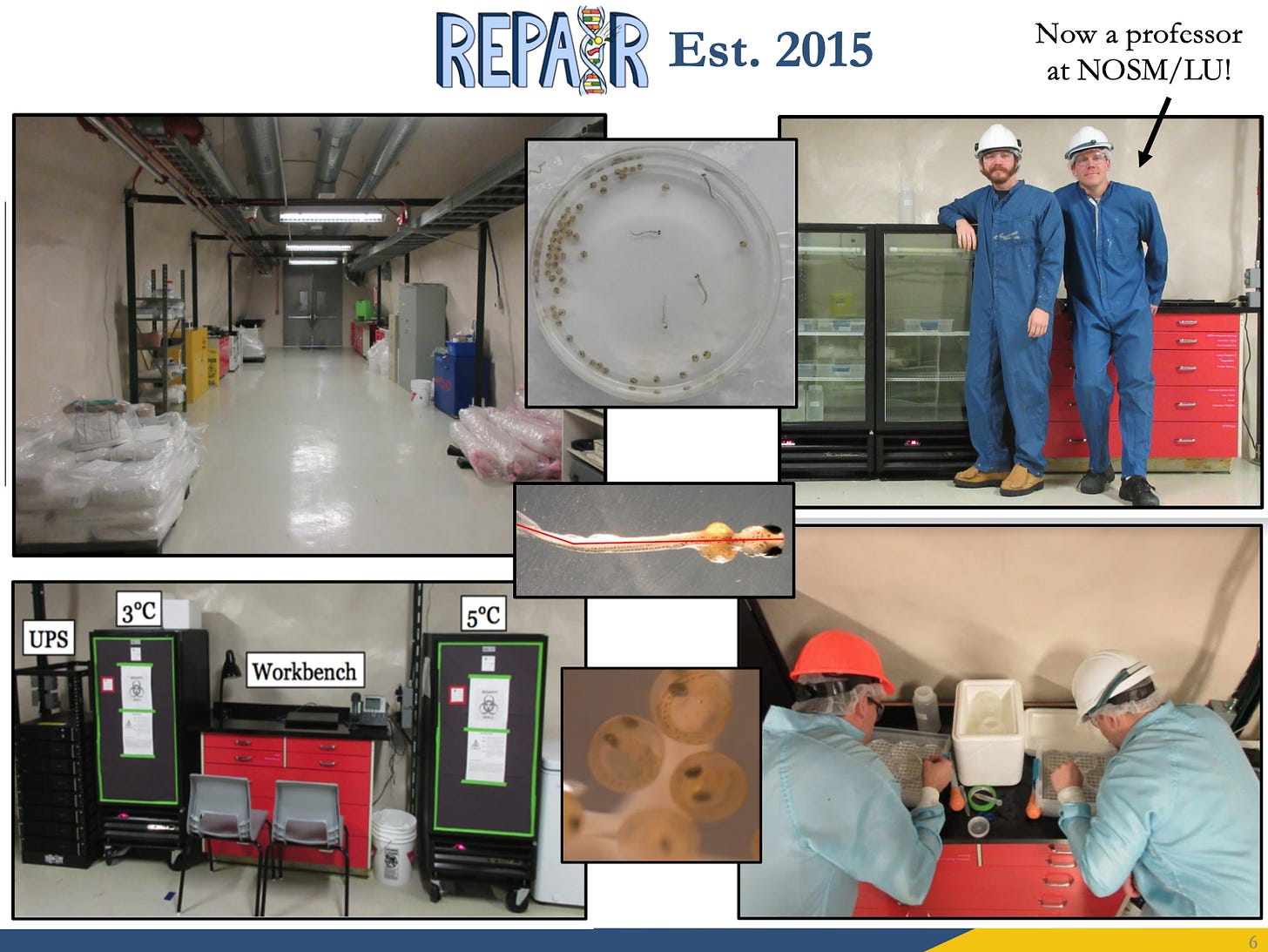
We're doing some work with yeast as well. We can actually take bakers yeast or brewers yeast and culture them underground in SNOLAB. We've also done work where we can desiccate them. So when you buy yeast at the grocery store where it's in a dried desiccated state. We can similarly desiccate our yeast strains and store them long-term down in the SNOLAB. So they're still alive but not actively growing. That way they're accumulating that radiation damage, or lack of damage. And we’re seeing some interesting things with the yeast. Again, this is all preliminary data, we're still following up on some of these studies. But we have seen that the yeast actually survive better in the presence of background radiation – so up on the surface – compared to when they're cultured down in SNOLAB.
We've also seen some interesting findings with human cell cultures. We see changes in some genes that we know are related to carcinogenesis. Potentially, that could mean we see an increase in mutation and carcinogenic frequency in the absence of background radiation. Again, it's preliminary data now, but that's what our results are starting to show.
MH: That's really interesting. You're studying yeast and human cells. If you zoom out, is there a way to design these experiments to reveal how low-doses of ionizing radiation affects life at the more macro human level? How do we translate what is happening in yeast or human cells to how the absence of background radiation affects humans and our diagnoses and treatments.
CT: That's always kind of challenging, especially with these types of experiments. Because in a lab like SNOLAB, we can be limited in terms of access. This limits us in what model systems we can use.
Yeast in particular, are genetically very similar to humans. And so if we're looking at genetic effects of these different radiation environments, we can actually infer a fair bit about what happens in humans based on the yeast systems.
We are moving into some more complex model systems. We've done some early experiments working with fruit flies. That's something that we're going to look at continuing in the future. We've also done some work on C. elegans, which is like a nematode worm.

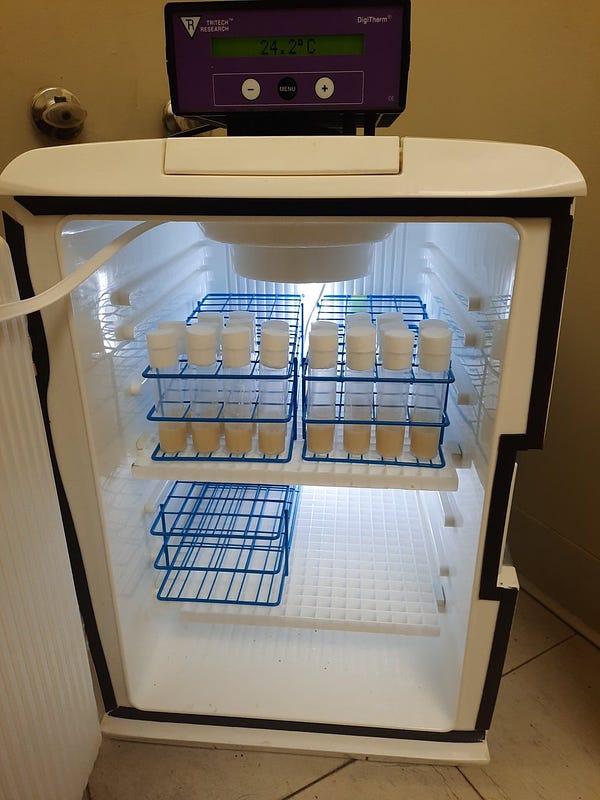
MH: Got it. You said you're in the process of analyzing the data and really getting to some findings. But have you had any sort of response to your research or findings yet? What has that been so far?
CT: We’ve published some preliminary papers. And hopefully in the next few months, we'll have the first main data publications from our experiments coming out. But we have presented this research at scientific conferences and some industry workshops, and we've got some pretty positive responses from people.
It's just a really unique environment to work in, and we're lucky that we have access to the facility. It's one of only a few in the world where you can do this type of research. I think people are generally interested because we are taking an innovative approach to answer the question of what happens when we're exposed to low-dose radiation. Is it harmful? Or is it beneficial? Or is there no risk? A lot of people are looking at radiation effects by exposing different systems to doses slightly above background radiation. We're doing the inverse now where we can remove background radiation and see what happens.
MH: As a nuclear advocate, I've attended radiation conferences and seminars. There seems to be this very active and contentious debate in the scientific community on the shape of the radiation risk profile, particularly with respect to low-doses of radiation. Are there people that consider your work controversial? Are there other labs or researchers that are taking a different approach to reinforce LNT or to debunk hormesis?
CT: Like you said, it is a controversial area of research. There are a number of groups like ours that show that the risk is low or actually negative at these low doses. But there are groups that suggest the opposite – that all doses of radiation are harmful.
There's always going to be people that fight back on our results and the research, but that's why we're really careful in how we design our experiments. We're making sure that we're not biasing the results in any way. And all this goes through peer-reviewed publication as well.
We are one of the few labs in the world that can do this, but there are other groups that are doing this work on background radiation as well. There's a group in Italy at the Gran Sasso lab; there's a group in the US at WIPP, a nuclear waste facility. They are doing very similar experiments to what we're doing, and they're getting similar findings as well. So it's not just us that are finding these effects. Results are being reproduced by other groups around the world which lends to the validity of it. If you're having more than one research group in different labs at different locations around the world finding similar results, then it's hard to discredit those findings.
MH: This is obviously a very complex field of research and a complex question – one that's particularly difficult to answer given the nature of the question. I'm wondering, though, can both sides sort of be right? With LNT, the whole idea is that you don't have to be completely inundated with radiation to get a double strand DNA break. If for example a single alpha particle can cause a double strand DNA break, that can cause bad code, and that could lead to cancer way down the road. In that respect, LNT could be correct. But if there was no radiation, there could be a whole host of problems in and of itself. So is it possible that very low doses always pose some level of risk, but that risk is very small when compared to the risk of removing that low-level ionizing radiation?
CT: I think you kind of nailed it there, which is why we as a field haven't figured this out and why there are people still looking into low dose effects. It's not necessarily a cut and dry response. If radiation hormesis exists, it doesn't necessarily apply to all types of radiation, to all settings, to all endpoints you're looking at.
And you mentioned before about scaling this up to more complex model systems and to humans. The research that we do, and that a lot of scientists do, is with cell culture model systems or animal model systems that are genetically almost identical. But that's not the case when we're looking at humans. Even if we have a hormetic response to radiation, it might not necessarily apply to all individuals. You have people that just naturally based on genetics are more susceptible to radiation compared to others. Maybe this is a response that only applies to certain people, and not all humans. I think especially when we're looking at human epidemiological data, that's where it gets a lot more complex to try to understand what's going on.
MH: I hadn't even thought about that – that there may be people who are more susceptible or people who are more resistant to radiation. That’s fascinating. So other labs are doing this research and are getting similar findings. How would you describe the current evidence for hormesis in humans? Would you say it's weak, speculative, emerging but promising, etc.?
CT: I wouldn't say it's new. The hormetic response is something that's been known for decades now. But the problem is figuring out what it applies to and how universal the response is. There are many groups out there that've shown hormetic responses in different model systems – whether we're talking growing cells in a dish, or whether we're talking rodent models or even in human models. But at the same time, there are other groups that have shown that those responses do not exist. I think the hormetic response does exist and enough different, unique groups have shown that we can get those responses.
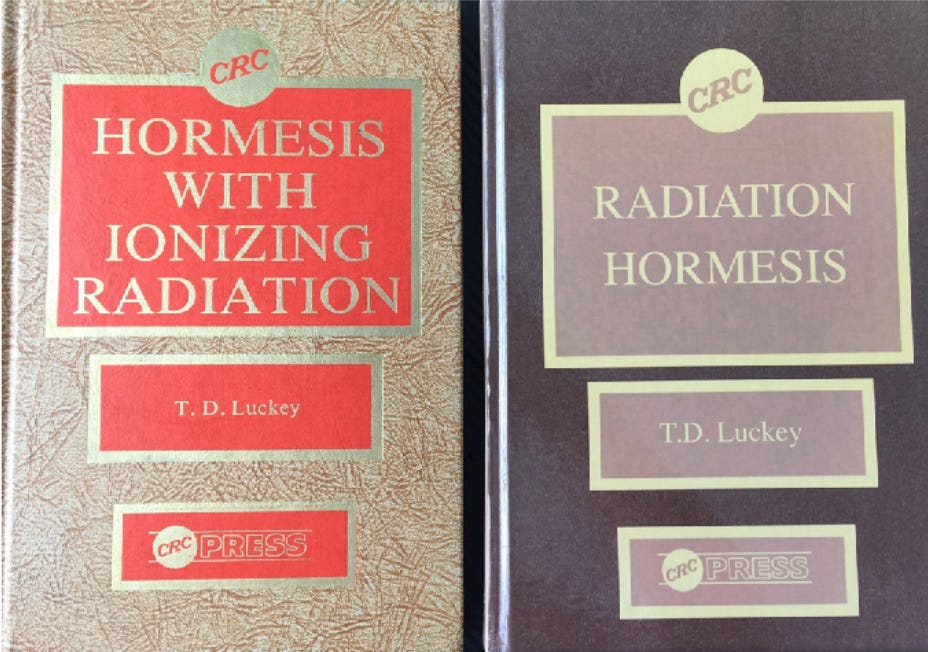
But it's probably not necessarily a universal response. So it's trying to figure out the types of radiation, doses and the dose rates where we can actually see that response. That's really what we're still trying to tease out.
MH: So what's next? I know you said you've got some upcoming data publications, but what's on the docket for the REPAIR project? What are you most excited about going forward?
CT: The work that's coming out is our first full experimental data where we're seeing some really interesting findings. Based on that, we're in the process now of designing the next set of experiments that will allow us to kind of dive a little deeper into some of those cool, interesting findings that we had in the first round of experiments.
We're going to continue to work with the human cells and the yeast to tease out some of those early findings, then we're also going to move into more complex model systems. I already mentioned the C elegans as well as fruit flies. That'll allow us to see if the responses we observed in the single cell model systems apply to the more complex systems as well.
It's kind of a combination of extending on what we've already done with the single cell model systems, and then transitioning somewhat to some of the more complex model systems.
MH: Awesome. I find SNOWLAB really fascinating in general, as well as the hormesis question and the way you're approaching it.
CT: We're lucky that we have SNOLAB. The setup we have there, with the additional shielding and radon control, is giving us one of the lowest, if not the lowest, background radiation dose rate in the world. Applying a reduction of potassium-40 is something that hasn't really been done by other research groups, and that can get us down to hundreds of fold difference from natural background levels. I think that's what's really going to be interesting – when we can show that not only have we removed almost all of the exogenous natural sources, but we're even able to significantly reduce those internal sources and really get down to a dose rate that is well-below anything that has ever been looked at before.
MH: Okay, last question. If you have the world's attention for 60 seconds, what would be your message about your work or radiation?
CT: Well, I would just say that low dose radiation isn't something that we need to be concerned about. We know that high doses of radiation are damaging, but that's not what humans are exposed to on a daily basis. There's this debate about hormesis vs threshold vs LNT models, but ultimately, the risk from low-dose radiation is exceptionally low regardless of which of those models you prescribe to.
When you put it in perspective, compared to other stresses we’re exposed to, radiation is actually a fairly poor carcinogen. If you're prescribed a CT scan, or if you're someone who works in a job where you get exposed to low doses of radiation, you should not be worried about those doses. Ultimately, when we use radiation in medicine, the benefit of those scans and those diagnostic procedures, in my opinion, far outweighs the risk of the radiation exposure.